Hydraulic hose assemblies are essential components in hydraulic transmission systems. The quality of the hose assembly directly affects the system and equipment performance. Therefore, it is necessary to establish testing methods and standards for hydraulic hose assemblies to ensure their reliability and longevity.
Definitions of Hydraulic Hose Assembly Testing Methods Parameters
Maximum Working Pressure
The Maximum Working Pressure (MWP) refers to the highest pressure at which the hydraulic hose assembly can safely operate under normal working conditions, without compromising system performance or safety. This is a critical specification, as exceeding the maximum working pressure can lead to hose failure, leakage, or rupture, potentially causing severe damage to the hydraulic system or machinery. The MWP is typically determined by the hose’s construction, materials, and reinforcement layers, ensuring that it can withstand the expected operating pressures without risk of failure.
For example, a hydraulic system designed to operate at 200 bar should use hose assemblies rated with a maximum working pressure that matches or exceeds this value. It’s essential for users to verify the hose assembly’s MWP to ensure it aligns with system pressure requirements.
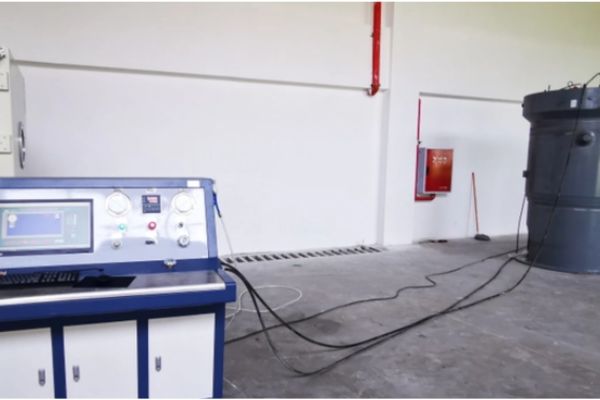
Length Change
Length Change refers to the axial elongation or shortening of the hydraulic hose assembly when subjected to its maximum working pressure. Under pressure, the hose material may expand or contract slightly, and this change is usually measured in millimeters or inches.
The amount of length change is important because it can affect the flexibility and positioning of the hose within the system. A hose assembly that elongates excessively may create additional strain on connectors, causing misalignments or even failures over time. Therefore, the permissible length change is strictly controlled and should be within manufacturer specifications to maintain optimal system performance.
Burst Pressure
The Burst Pressure is the pressure at which the hydraulic hose assembly will fail or rupture. It is typically measured at a pressure level that is twice the maximum working pressure. The burst pressure test ensures that the hose can handle extreme surges or unexpected pressure spikes that may occur during operation.
Hose assemblies with higher burst pressure ratings are generally considered safer and more durable, as they can withstand conditions beyond normal operating pressures without catastrophic failure. However, relying on burst pressure as a safeguard is not advisable, as the system should always operate within the maximum working pressure to ensure reliability.
For instance, if a hydraulic hose is rated for a maximum working pressure of 150 bar, its burst pressure should be at least 300 bar to ensure it can endure temporary pressure spikes or overloads without bursting.
Minimum Burst Pressure
The Minimum Burst Pressure is defined as the lowest pressure at which the hose assembly will fail. It is typically set at four times the maximum working pressure. This high safety margin ensures that even in the case of a sudden pressure surge or system malfunction, the hose assembly can withstand the pressure without immediate catastrophic failure.
For example, for a hose rated for 100 bar MWP, the minimum burst pressure would be 400 bar. This substantial difference between working and burst pressures ensures that the hose can provide extra protection in case of unexpected pressure conditions, such as hydraulic shock, without compromising the safety of the system.
Pulsation
Pulsation refers to the cyclical or rapid fluctuations in pressure that can occur within the hydraulic system, often caused by variable flow or the action of hydraulic pumps. Pulsation is typically characterized by rapid increases and decreases in pressure, which can place significant strain on hoses, fittings, and other components.
Hydraulic hoses must be designed to withstand pulsations without fatigue or failure. Pulsations can lead to hose fatigue, causing the material to degrade over time or leading to internal hose wear. The ability of a hydraulic hose assembly to endure these cyclical pressure changes without failure is crucial to maintaining system performance and longevity.
In practical terms, pulsation testing ensures that the hose can handle rapid pressure variations without showing signs of failure, such as cracking or bursting. Pulsation resistance is an essential aspect of hose design for applications like pumps, valves, and hydraulic cylinders that often experience cyclic pressure fluctuations.
Pressure Endurance Test
The Pressure Endurance Test is a crucial evaluation to assess the durability and integrity of a hydraulic hose assembly when exposed to high pressures. In this test, the hose assembly is subjected to a static pressure that is twice its rated maximum working pressure. The purpose of the test is to determine whether the hose can withstand extreme pressure conditions without failing and to ensure that it can maintain its structural integrity under operational stress.
Test Objective: The hydraulic hose assembly should be able to withstand the test pressure for a minimum of 60 seconds without exhibiting signs of failure, such as deformation, leakage, or rupture. This ensures that the hose can perform safely and effectively even under high-pressure conditions that may occur during certain operational scenarios or system malfunctions.
Test Procedure
Preparation:
Connect the Hose Assembly: The hydraulic hose assembly is securely connected to a hydraulic pressure source that can generate the required test pressure.
Pressure Gauge Installation: A calibrated pressure gauge is installed to monitor the pressure being applied to the hose assembly, ensuring that the pressure is increased gradually and accurately.
Gradual Pressure Increase:
The pressure is increased slowly to avoid sudden shocks to the hose. This helps prevent any immediate rupture or damage due to abrupt pressurization.
The pressure should be increased until it reaches 2 times the maximum working pressure (i.e., double the rated pressure of the hose). For example, if the maximum working pressure is 200 bar, the test pressure will be set to 400 bar.
Holding at Test Pressure:
The assembly must remain at this elevated pressure for at least 60 seconds (or longer, depending on the standard being applied).
During this time, the hose assembly is carefully monitored for any signs of failure, such as:
Deformation: Any permanent change in shape, such as elongation or bulging, indicating the hose’s inability to maintain its structural integrity.
Leakage: Any fluid leakage from the hose or fittings, which could indicate weaknesses in the hose material or connections.
Rupture: The most severe failure, where the hose bursts or tears, potentially causing system malfunction or safety hazards.
Post-Test Inspection:
After the 60-second duration, the pressure is gradually released, and the hose assembly is visually inspected for any permanent deformation, leakage, or rupture.
The hose should not exhibit any permanent changes in shape or performance. If there are signs of failure, the assembly fails the test and would need to be replaced or re-engineered.
Evaluation Criteria
Pass: The hose assembly withstands the pressure for 60 seconds without any signs of permanent deformation, leakage, or rupture. It is deemed suitable for use under high-pressure conditions.
Fail: If the hose shows any signs of damage or failure, such as leaks, bursts, or permanent deformations, it fails the test and is considered unfit for the intended application.
Importance of the Pressure Endurance Test
The Pressure Endurance Test is essential for ensuring that hydraulic hoses meet industry standards for safety and performance. By exposing the hose assembly to pressures beyond its rated capacity, this test simulates extreme conditions that the system may encounter during operation or under malfunction scenarios. It helps identify potential weaknesses in the hose’s construction, material, or connections, thereby improving the overall reliability and safety of the hydraulic system.
This test also plays a key role in the quality control process for manufacturers, providing assurance that the hoses they produce will perform safely and effectively under high-pressure conditions in the field.
Burst Pressure Test
The Burst Pressure Test is a critical test used to determine the maximum pressure a hydraulic hose assembly can handle before it fails. This test is designed to ensure the hose can withstand extreme pressure conditions well beyond its normal operating range, thus providing an added layer of safety for the system. The burst pressure is typically four times the hose assembly’s maximum working pressure. If the hose can endure this extreme pressure without failure, it demonstrates superior strength and reliability under high-stress conditions.
Test Objective: The Burst Pressure Test assesses the hose’s ability to withstand a much higher pressure than it would normally encounter in operation, simulating a worst-case scenario where sudden pressure surges or system failures occur. By evaluating the hose’s performance under these extreme conditions, the test ensures that the hose will not fail prematurely, even under unexpected high-pressure events.
Test Procedure
Preparation:
Connect the Hose Assembly: The hydraulic hose assembly is securely connected to a pressure source capable of providing controlled, gradually increasing pressure.
Install Pressure Monitoring Devices: A reliable pressure gauge or sensor is used to monitor the pressure applied to the hose, ensuring precise measurement as the pressure increases.
Gradual Pressure Increase:
The pressure is increased slowly, typically starting from the normal operating pressure level, until it reaches four times the maximum working pressure of the hose. This gradual increase is essential to avoid abrupt pressure spikes that could prematurely damage the hose.
The pressure is increased in stages to carefully monitor the hose’s response to each incremental increase.
Monitoring for Failure:
Throughout the process, the hose assembly is closely monitored for any signs of failure, such as visible bulging, deformation, or ruptures. This is a crucial observation point, as the test will continue until the hose eventually bursts.
Once the hose assembly bursts, the exact pressure at the moment of rupture is recorded. This value is considered burst pressure, and it provides valuable data on the hose’s strength and ability to withstand extreme pressure levels.
Post-Test Evaluation:
After the test is complete, the burst pressure and the point of failure are analyzed. The goal is to ensure that the hose assembly did not fail below the expected burst pressure threshold (i.e., four times the maximum working pressure).
Any observed failures are documented, and the hose’s material quality, design, and construction are evaluated to identify any weaknesses or areas for improvement.
Importance of the Test: The Burst Pressure Test is an essential measure of the safety margin for hydraulic hose assemblies. By confirming that a hose can handle pressures significantly higher than its rated maximum working pressure, manufacturers can ensure that their hoses will perform reliably and safely even in the event of unexpected pressure surges or system faults. This test also provides assurance to end-users that the hose assembly is built to withstand extreme conditions and is unlikely to fail under normal or slightly higher-than-normal operational pressures.
Pulsation Test
The Pulsation Test is designed to simulate the pressure fluctuations that are commonly experienced in hydraulic systems during operation. In real-world applications, hydraulic systems often experience rapid and repetitive changes in pressure, such as during the activation and deactivation of valves, or due to load variations in the system. The pulsation test ensures that the hydraulic hose assembly can maintain its structural integrity and function reliably under these dynamic, cyclic pressure conditions.
Test Objective: The primary goal of the Pulsation Test is to evaluate the hose assembly’s ability to withstand rapid and repeated changes in pressure without failure. This includes checking for issues such as leaks, material fatigue, deformations, or rupture that may occur due to the cyclical nature of hydraulic pressure.
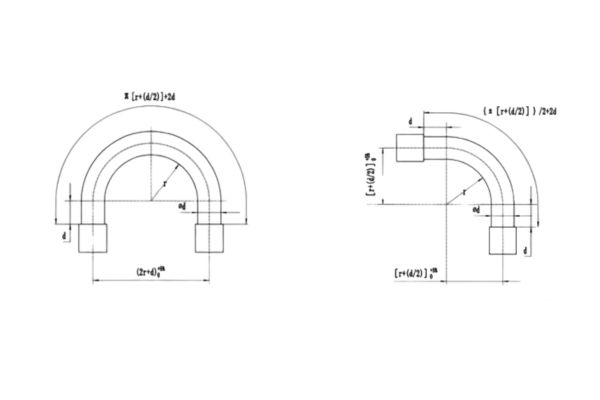
Test Procedure
Preparation:
Connect the Hose Assembly: The hydraulic hose assembly is connected to a pulsation rig or test bench that can generate rapid pressure cycles, simulating the fluctuating pressure conditions experienced in a working hydraulic system.
Set Pressure Parameters: The pressure levels should be set within the rated working pressure of the hose assembly, ensuring that the test reflects real-world operating conditions. The pressure will fluctuate between a minimum and maximum value according to the system’s design.
Application of Pressure Cycles:
The hose assembly is subjected to rapid pressure cycles, where the pressure is repeatedly increased and decreased, simulating the normal operation of hydraulic systems (e.g., the opening and closing of valves or operating machinery under varying loads).
Cycle Duration and Count: The test typically involves applying a large number of cycles—often thousands or more—depending on the specific requirements of the hose assembly. The number of cycles is recorded to ensure that the hose assembly can endure prolonged pressure fluctuations.
Monitoring for Failure Signs:
Throughout the test, any signs of failure, such as leaks, deformations, or ruptures, are carefully observed. These failures may occur due to the cumulative effects of pressure fluctuations over time, which can lead to material fatigue or weak points in the hose assembly.
The pressure levels and cycle counts are monitored to ensure the hose is subjected to the correct conditions, and any premature signs of damage are noted.
Evaluation:
Once the pulsation test is completed, the hose assembly is evaluated for its performance. If no significant issues such as leaks, cracks, or pressure loss are observed, the hose has passed the test. The ability to withstand a high number of pressure cycles without failure indicates that the hose is durable and reliable under dynamic working conditions.
Key Considerations:
The pulsation frequency (the speed of the pressure cycles) and the pressure range used in the test should mimic the actual operating conditions of the hydraulic system in which the hose assembly will be used.
The test duration can vary depending on the intended application, with some hose assemblies required to endure hundreds of thousands or even millions of cycles before being deemed suitable for use.
Length Change Test
The Length Change Test is designed to assess how much the hydraulic hose assembly’s length changes when exposed to the maximum working pressure. Hydraulic hoses are subjected to varying pressures during normal operation, and understanding how the hose responds to pressure is crucial for ensuring the system’s overall integrity. The length change is an important parameter that helps to determine the hose’s ability to maintain its flexibility, alignment, and functionality under pressure. Excessive length change can indicate potential issues with the hose’s reinforcement or flexibility, which may affect system performance.
Test Objective: The test measures the axial elongation or contraction of the hose under its maximum working pressure to ensure that the hose assembly maintains its designed dimensions and does not exhibit excessive deformation. A significant change in length may lead to issues like misalignment, strain on fittings, and compromised system performance.
Test Procedure
Preparation:
Secure the Hose Assembly: The hydraulic hose assembly is securely installed and connected to a pressure source that can generate the required maximum working pressure.
Measure Initial Length: Before applying pressure, the axial length of the hose is carefully measured using accurate measuring tools. This measurement serves as the baseline for comparison.
Pressurization:
The hose is pressurized gradually to its maximum working pressure. This pressure should be consistent with the hose’s rated maximum working pressure, as defined in the hose specifications.
Measure Post-Test Length:
After the hose assembly has reached the maximum working pressure, the axial length is measured again to determine how much the hose has expanded or contracted. This measurement is compared to the original length to calculate the total length change.
Analysis:
The change in length is then compared against the manufacturer’s acceptable tolerances. A minimal, controlled change in length is expected, but excessive elongation or contraction may indicate an issue with the hose’s structural integrity, such as weakening reinforcement or issues with the hose material.
Evaluation:
If the length change falls within the specified limits, the hose passes the test. However, if the length change exceeds acceptable tolerances, it may indicate a potential problem, such as poor hose construction or inappropriate material choices, and further investigation or adjustments are required.
Conclusion
Testing hydraulic hose assemblies is essential for ensuring the reliability and safety of hydraulic systems. By conducting these tests, such as pressure endurance, burst pressure, pulsation, and length change tests, manufacturers can verify that their hose assemblies meet the required standards for durability and performance. Regular testing also helps prevent system failures, reduces maintenance costs, and improves overall equipment longevity.
FAQ
What is the purpose of the Pressure Endurance Test for hydraulic hoses?
The Pressure Endurance Test checks if the hydraulic hose can withstand twice its maximum working pressure for at least 60 seconds without signs of deformation or leakage.
How is the Burst Pressure Test conducted?
The Burst Pressure Test subjects the hose to a pressure that is four times its maximum working pressure until the hose fails. This test ensures the hose can handle extreme pressure conditions.
What is a pulsation test and why is it important?
The pulsation test simulates rapid pressure cycles that occur in hydraulic systems, ensuring the hose assembly can withstand these fluctuations without failure, such as leaks or deformations.
What is the Length Change Test?
The Length Change Test measures the axial expansion or contraction of the hose when subjected to its maximum working pressure, ensuring the hose maintains its dimensional stability during operation.
Why is regular testing of hydraulic hoses necessary?
Regular testing ensures that the hose assemblies can perform under various conditions and pressures, preventing system failures, extending hose life, and ensuring operational safety.
What signs indicate a failure during the tests?
Signs of failure include hose rupture, leakage, excessive deformation, or loss of structural integrity. These issues suggest that the hose may not meet the required safety or performance standards.