Hydraulic systems are the backbone of countless industrial, mobile, and aerospace applications, providing the power and precision necessary for critical operations. From heavy machinery operating in scorching deserts to aircraft flying through frigid stratospheres, these systems rely on the integrity of their components to function reliably. Among these, hydraulic hoses and their connector seals are paramount, acting as the lifelines that transmit fluid power. However, their performance is acutely sensitive to temperature, an often-underestimated factor that can lead to premature failure, costly downtime, and even catastrophic safety incidents. This article, aimed at extreme environment application engineers and material scientists, delves into the intricate relationship between temperature and hydraulic hose performance, offering insights into degradation mechanisms, material science advancements, and practical mitigation strategies.
The Unseen Enemy: Temperature’s Impact on Hydraulic Hose Performance
Temperature is a pervasive and often insidious factor that significantly influences the performance and longevity of hydraulic hoses. Unlike immediate mechanical stresses, thermal degradation can be a gradual process, silently compromising the hose’s structural integrity until a sudden and often catastrophic failure occurs. Both excessively high and extremely low temperatures, as well as rapid fluctuations between them, pose unique challenges to the polymeric and elastomeric materials that constitute hydraulic hoses.
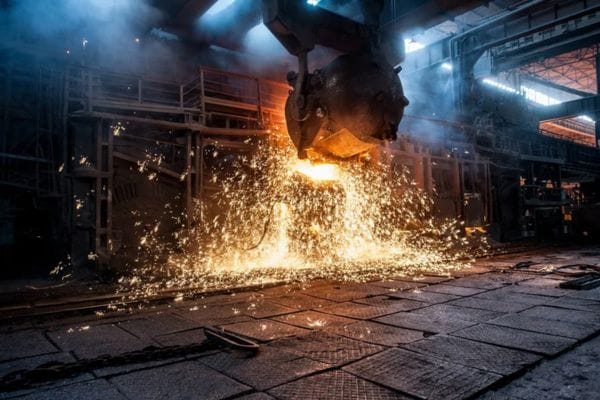
High-Temperature Aging: The Silent Degradation
High temperatures accelerate chemical reactions within the hose materials, leading to a phenomenon known as thermal aging. This process primarily affects the elastomeric compounds used in the inner tube and outer cover, as well as the adhesive layers bonding the reinforcement plies. At elevated temperatures, polymer chains can undergo several detrimental changes:
- Oxidation: Exposure to heat and oxygen causes the polymer chains to react, leading to chain scission (breaking of molecular bonds) or cross-linking (formation of new bonds). Both processes alter the material’s molecular structure, resulting in a loss of elasticity, increased hardness, and reduced tensile strength. The hose becomes brittle and prone to cracking, especially under dynamic stress.
- Plasticizer Leaching: Many elastomeric compounds contain plasticizers, additives that enhance flexibility and workability. Prolonged exposure to high temperatures can cause these plasticizers to migrate out of the material, leading to a significant increase in hardness and a reduction in flexibility. This makes the hose stiff and susceptible to cracking, particularly at bends or points of vibration.
- Volatilization: Some components of the hose material, including certain additives or unreacted monomers, can volatilize (evaporate) at high temperatures. This loss of material can lead to shrinkage, embrittlement, and a reduction in the hose’s overall volume and structural integrity.
- Fluid Degradation: The hydraulic fluid itself can degrade at high temperatures, forming acidic byproducts, sludge, and varnish. These degraded fluids can chemically attack the inner tube material, further accelerating its deterioration and potentially leading to blockages within the system.
Low-Temperature Hardening: Brittleness and Failure
Conversely, extremely low temperatures present a different set of challenges for hydraulic hoses, primarily leading to a loss of flexibility and an increase in brittleness. This phenomenon, often referred to as low-temperature hardening or embrittlement, occurs as the elastomeric materials transition from a flexible, rubbery state to a rigid, glassy state. The key mechanisms include:
- Glass Transition: Polymers have a characteristic glass transition temperature (Tg), below which they become hard and brittle. As the ambient or fluid temperature drops below the hose material’s Tg, the molecular chains lose their mobility, making the material rigid and susceptible to cracking under stress or impact. This is particularly problematic in applications where hoses are subjected to bending, vibration, or pressure impulses in cold conditions.
- Crystallization: Some polymers can undergo crystallization at low temperatures, where molecular chains align into ordered structures. This process further increases the material’s stiffness and reduces its flexibility, making it more prone to fracture.
- Fluid Viscosity Increase: At low temperatures, hydraulic fluids become significantly more viscous. This increased viscosity can lead to higher pressure drops, reduced flow efficiency, and increased strain on the hose assembly, especially during cold starts. The combination of a stiff hose and high-viscosity fluid can exacerbate the risk of hose failure.
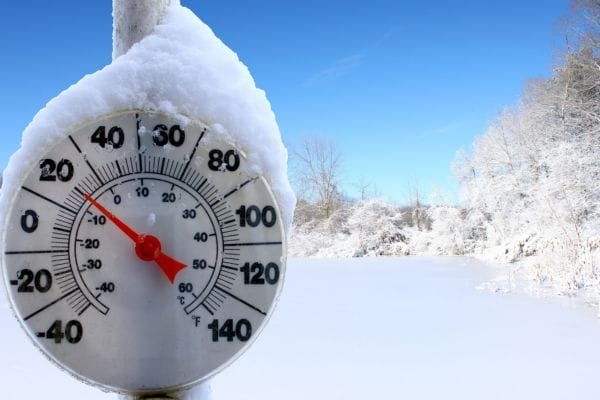
Thermal Shock: The Sudden Destroyer
Thermal shock occurs when a hydraulic hose experiences a rapid and significant change in temperature, either from hot to cold or cold to hot. This sudden temperature differential creates internal stresses within the hose materials due to differential thermal expansion and contraction. While high-temperature aging and low-temperature hardening are gradual processes, thermal shock can lead to immediate and catastrophic failure. The primary mechanisms involved are:
- Differential Thermal Expansion/Contraction: Different layers of the hydraulic hose (inner tube, reinforcement, outer cover) are made from different materials with varying coefficients of thermal expansion. A rapid temperature change causes these layers to expand or contract at different rates, leading to internal shear stresses at the interfaces between layers. If these stresses exceed the material’s strength, delamination, cracking, or blistering can occur.
- Material Fatigue: Repeated thermal shock cycles can induce fatigue in the hose materials. Even if immediate failure does not occur, the cumulative effect of these stresses can lead to microscopic cracks that propagate over time, eventually resulting in macroscopic failure. This is particularly relevant in applications with intermittent operation or exposure to rapidly changing environmental conditions.
- Fluid Property Changes: Rapid temperature changes also affect the hydraulic fluid’s viscosity and density, leading to sudden pressure fluctuations within the hose. This dynamic pressure, combined with the material stresses from thermal expansion/contraction, further increases the risk of failure.
Engineering for Extremes: Hydraulic Hose Temperature Ratings and Technical Parameters
Hydraulic hoses are designed and manufactured to meet specific performance standards, with temperature ratings being a critical parameter. These ratings define the safe operating temperature range for both the hydraulic fluid and the ambient environment. Exceeding these limits, even intermittently, can drastically reduce the hose’s lifespan and compromise system safety. Manufacturers typically provide a temperature range for their hoses, often specified in both Celsius and Fahrenheit.
For example, a standard hydraulic hose might be rated for -40°C to +100°C (-40°F to +212°F). However, for extreme applications, specialized hoses are available with much wider temperature ranges. These hoses often incorporate advanced materials and construction techniques to withstand more severe thermal conditions. Key technical parameters related to temperature include:
- Operating Temperature Range: This is the most fundamental parameter, indicating the continuous temperature range within which the hose is designed to operate without significant degradation. It typically covers both the fluid temperature and the ambient temperature.
- Fluid Compatibility at Temperature: The chemical compatibility between the hose’s inner tube material and the hydraulic fluid can change with temperature. Manufacturers specify the types of fluids compatible with the hose at its rated temperature range. For instance, a hose suitable for petroleum-based fluids at 100°C might not be suitable for water-glycol fluids at the same temperature.
- Impulse Cycle Life at Temperature: Hydraulic hoses are often subjected to pulsating pressures. The impulse cycle life, or the number of pressure cycles a hose can withstand before failure, is significantly affected by temperature. Higher temperatures generally reduce the impulse cycle life. Manufacturers may provide impulse ratings at different temperatures to reflect this.
- Low-Temperature Flexibility: This parameter quantifies the hose’s ability to remain flexible at low temperatures. It is crucial for applications where hoses are routed in tight spaces or subjected to movement in cold environments. Tests often involve bending the hose at a specified low temperature and checking for cracks or damage.
- High-Temperature Resistance: This refers to the hose’s ability to resist degradation, hardening, and embrittlement at elevated temperatures. It is often evaluated through accelerated aging tests where the hose is exposed to high temperatures for extended periods, followed by performance evaluations.
To illustrate the variation in temperature ratings, consider the following table, which provides a generalized overview of common hydraulic hose types and their typical temperature ranges. It’s important to note that these are general guidelines, and specific product specifications from manufacturers should always be consulted.
Hose Type | Inner Tube Material | Reinforcement Material | Outer Cover Material | Typical Temperature Range (°C) | Typical Temperature Range (°F) |
Standard Rubber | NBR (Nitrile) | Steel Wire | Synthetic Rubber | -40 to +100 | -40 to +212 |
High-Temp Rubber | HNBR (Hydrogenated Nitrile) | Steel Wire | Synthetic Rubber | -40 to +135 | -40 to +275 |
PTFE (Teflon) | PTFE | Stainless Steel Braid | PTFE or Braided | -60 to +260 | -75 to +500 |
Thermoplastic | Polyester/Polyurethane | Synthetic Fiber/Wire | Polyurethane | -40 to +93 | -40 to +200 |
Low-Temp Rubber | Specialty NBR/EPDM | Steel Wire | Synthetic Rubber | -55 to +100 | -67 to +212 |
Note: These ranges are approximate and can vary significantly based on specific manufacturer, construction, and application. Always refer to the manufacturer’s data sheet for precise specifications.
Material Science at Work: Advanced Materials for Extreme Temperature Hoses
The ability of hydraulic hoses to perform reliably in extreme temperatures is fundamentally linked to advancements in material science. Traditional rubber compounds, while suitable for many applications, fall short when faced with continuous exposure to very high or very low temperatures, or rapid thermal cycling. Engineers and material scientists have developed a range of advanced materials and composite structures to overcome these limitations.
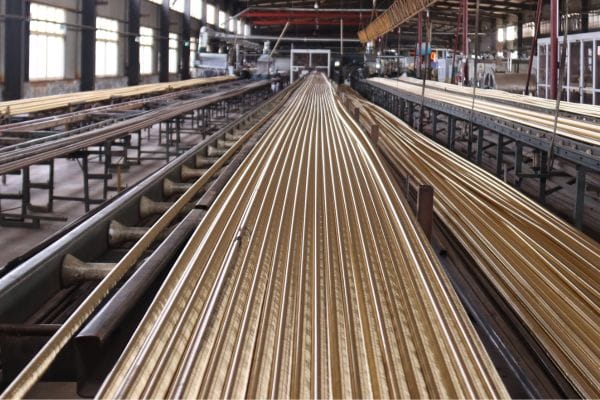
Elastomers for High Temperatures
- Hydrogenated Nitrile Butadiene Rubber (HNBR): HNBR offers improved heat resistance compared to standard NBR, with continuous operating temperatures up to 150°C (302°F). Its enhanced thermal stability and resistance to oxidation make it suitable for many high-temperature hydraulic applications.
- Fluoroelastomers (FKM/Viton): FKM, commonly known by the brand name Viton, provides excellent heat resistance, with continuous operating temperatures up to 200°C (392°F) and intermittent exposure to even higher temperatures. FKM also offers superior chemical resistance, making it ideal for applications involving aggressive hydraulic fluids at high temperatures.
- Perfluoroelastomers (FFKM): For the most extreme high-temperature and chemical resistance requirements, FFKM materials are employed. These materials can withstand continuous temperatures up to 300°C (572°F) and are virtually inert to most chemicals, making them suitable for highly specialized and critical applications.
Elastomers for Low Temperatures
- Specialty Nitrile Compounds: While standard NBR has a Tg around -40°C, specialized NBR compounds can be formulated with lower glass transition temperatures, extending their flexibility down to -55°C (-67°F) or even lower. These formulations often involve different acrylonitrile content or the addition of specific plasticizers.
- Ethylene Propylene Diene Monomer (EPDM): EPDM elastomers offer excellent low-temperature flexibility, with a Tg typically below -50°C (-58°F). They are also resistant to water-based fluids and some synthetic hydraulic fluids, making them suitable for certain cold-weather applications.
- Silicone Rubber: Silicone rubber exhibits exceptional flexibility at very low temperatures, with some grades maintaining properties down to -100°C (-148°F). However, silicone typically has lower tensile strength and abrasion resistance compared to other elastomers, limiting its use in high-pressure hydraulic hoses.
Thermoplastics and Fluoropolymers
- Polytetrafluoroethylene (PTFE): PTFE, often referred to as Teflon, is a fluoropolymer renowned for its extremely wide operating temperature range, typically from -60°C to +260°C (-75°F to +500°F). It also offers excellent chemical inertness and a very low coefficient of friction. PTFE hoses are often reinforced with stainless steel braids to handle high pressures and are a preferred choice for applications requiring both extreme temperature and chemical resistance.
- Thermoplastic Elastomers (TPEs): TPEs combine the processing advantages of thermoplastics with the elastic properties of rubbers. Certain TPE formulations can offer good flexibility at low temperatures and improved heat resistance compared to standard thermoplastics, making them suitable for specific hydraulic hose applications.
Reinforcement Materials
Beyond the inner tube and outer cover, the reinforcement layers are crucial for the hose’s pressure rating and structural integrity at extreme temperatures. While steel wire braids and spirals are common, specialized materials are used for enhanced performance:
- High-Tensile Steel Wire: For high-pressure applications at elevated temperatures, high-tensile steel wire with improved fatigue resistance is used. The manufacturing process ensures that the wire retains its strength and integrity even when subjected to thermal stress.
- Synthetic Fibers: For lighter-weight or more flexible hoses, synthetic fibers like aramid (e.g., Kevlar) or polyester are used. These materials offer good strength-to-weight ratios and can be engineered for specific temperature resistances.
If you’re engineering for temperature extremes, now’s the time to rethink your hose strategy. Contact Topa today to get expert support and custom solutions that keep your systems running, no matter the conditions.
FAQ
What is the primary cause of hydraulic hose failure in high temperatures?
High temperatures accelerate material degradation through oxidation and plasticizer leaching, leading to hardening and cracking.
How does low temperature affect hydraulic hoses?
Low temperatures cause hoses to become brittle and lose flexibility, making them prone to cracking and rupture.
What is thermal shock in hydraulic hoses?
Thermal shock is rapid temperature change causing internal stresses and potential delamination or cracking.
Are all hydraulic hoses suitable for extreme temperatures?
No, specialized hoses with advanced materials are required for extreme high or low temperature applications.
How can I extend the life of hydraulic hoses in extreme heat?
Use high-temperature rated hoses, protective sleeves, and ensure proper fluid cooling.
What should I do if my hydraulic hose freezes?
Do not bend or operate the hose; allow it to thaw naturally or with gentle warming before use.